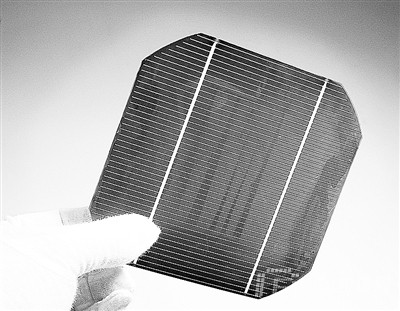
Silicon (Si) -based technologies have dominated the semiconductor industry over the past few decades. However, in recent years, there have been signs of a shift to more advanced materials in the market, such as to gallium nitride (GaN) and silicon carbide (SiC). Today, Gans and SiC are making major leaps in performance and efficiency, and these advances are transforming multiple fields from consumer electronics to industrial power systems.
At the same time, purchasing departments also need to consider the cost, quality and availability of these materials, GaN and SiC semiconductors are currently facing high prices, high defect density, material supply chain constraints and other challenges, semiconductor manufacturers are actively addressing these issues.
Technical advantages of wide bandgap semiconductors
GaN and SiC have characteristics that make them superior to traditional silicon (Si), such as their wider band gap, which means higher breakdown voltage, better thermal stability, more stable reliability, and more efficient operation at high frequencies and high power. These characteristics make it particularly useful in power electronics applications, particularly in frequency converters and renewable energy systems, where the role of high efficiency and thermal management is critical.
On the one hand, because GaN has high electron mobility properties, it is well suited for high-frequency applications. Gans offer faster switching speeds, lower energy losses, and smaller device sizes, which make them particularly attractive in microwave applications, such as 5G telecommunications and radar systems.
On the other hand, the robustness of SiC at high voltages and temperatures makes it the semiconductor material of choice for high-power applications, such as electric vehicle (EV) powertrains, renewable energy systems and industrial machinery.
There are now many market forces driving the shift to GaN and SiC, with industries emphasizing the need to meet stringent environmental regulations and the pursuit of further reductions in operating costs, and there is a very high demand for energy efficient solutions. For the same or similar power conversion tasks, devices using GaN and SiC devices consume less energy than those using traditional materials, making them an attractive option for sustainable-focused industries.
Electric cars are a prime example of this shift. The characteristics of SiC can work at high temperature and high pressure, making the power electronic equipment using SiC devices more efficient, not only can extend the driving range of the car, but also shorten the charging time of the power battery. Today, automotive manufacturers and suppliers have taken note of these advantages and are increasingly integrating SIC-based devices into their designs.
Similarly, the excellent performance of GaN in high-frequency applications has also promoted its application process in the field of telecommunications. The deployment of 5G networks requires high frequency, high voltage and high efficiency of power devices. Gallium nitride semiconductors can work at high frequency and high voltage, and are becoming an indispensable part of the infrastructure supporting the next generation of wireless communication.
Other advances include the introduction of "nanoparticles" in GaN and SiC substrate development. A "nanoparticle" is a tiny particle, usually between 1 and 100 nanometers in size, also known as a "nanocrystal" because of its crystal structure. It is a cost-effective solution that can be used to produce a variety of economical devices, such as fuel cells and solar cells. Compared with bulk materials, nanoparticles have a higher surface area to volume ratio and can significantly improve the performance of the device.
This improves the efficiency of energy applications and reduces the consumption of materials. In the field of healthcare, "nanoparticle" technology brings superior functionality to drug delivery and diagnostic systems. As a result, "nanoparticle" technology can drive cost reduction and performance improvement in many areas.
Impact on manufacturing and procurement
Despite the many advantages of GaN and SiC semiconductors, they also face some challenges. Compared to traditional silicon-based materials, GaN and SiC materials are more complex to manufacture and require greater consistency - the availability of their substrates, defect density and epitaxial growth techniques are essential for ongoing research and development.
The high cost is the main procurement issue, GaN and SiC are significantly more expensive to produce than Si, mainly due to the complexity of their growth process. For example, the epitaxial growth required for high-quality GaN and SiC involves advanced technologies that are more costly than those used in Si.
In addition, the supply of large-size wafers is now limited, which will not only increase the cost of using advanced semiconductors, but also affect the scalability of the manufacturing process. At the same time, it may also hinder the adoption of GaN and SiC in some industries, especially those with thin margins.
Supply chain and quality control also pose additional challenges. GaN and SiC are still in their infancy, which means that there are fewer suppliers capable of consistently producing high-quality materials, potentially leading to capacity bottlenecks and extended lead times, complicating planning and production schedules for companies that rely on these components.
In addition, ensuring the reliability and sensitivity of the equipment requires strict quality control measures. Due to the high defect density of these materials, but also sensitive to impurities, their production needs to go through a rigorous testing and verification process, which also increases the complexity of the overall procurement.
Fortunately, the industry has made significant progress in addressing these challenges. Advances in volume production, epitaxial growth, and device fabrication have steadily reduced costs and improved the quality of gallium nitride and silicon carbide devices. In addition, with the realization of economies of scale, the price gap between GaN/SiC and Si is expected to narrow, and wide-band gap semiconductors will be more readily available in the future.
The development prospects of gallium nitride and silicon carbide are bright. The continued pursuit of energy efficiency, coupled with surging demand in industries such as electric vehicles and telecommunications, is likely to accelerate the adoption of these advanced materials. From semiconductor manufacturers to end-user/end-user industry stakeholders, there is a growing awareness of the value of GaN and SiC.
Integrate GaN and SiC into the supply chain
In short, the shift to GaN and SiC is significant for the semiconductor industry. Their superior electrical properties and the growing demand for efficient, high-performance electronic devices are driving this shift. While challenges remain, technological advances and clear market demand indicate that GaN and SiC will play an important role in the future of semiconductor technology.
The Products You May Be Interested In
![]() |
AISC-1210H-2R2N-T | FIXED IND 2.2UH 1.3A 124 MOHM | 471 More on Order |
![]() |
AMPDGGI-A02T3 | OSC MEMS XO DUAL OUTPUT | 229 More on Order |
![]() |
AMPMDDC-50.0000T3 | MEMS OSC XO 50.0000MHZ CMOS SMD | 312 More on Order |
![]() |
AMPMGFA-4.0000T | MEMS OSC XO 4.0000MHZ CMOS SMD | 307 More on Order |
![]() |
AMPMEFA-66.6660T | MEMS OSC XO 66.6660MHZ CMOS SMD | 163 More on Order |
![]() |
AMPMADA-14.7456T | MEMS OSC XO 14.7456MHZ CMOS SMD | 483 More on Order |
![]() |
AMPMEGB-44.0000 | MEMS OSC XO 44.0000MHZ CMOS SMD | 220 More on Order |
![]() |
AMPMEDB-3.5700 | MEMS OSC XO 3.5700MHZ CMOS SMD | 162 More on Order |
![]() |
ASTMUPCD-33-20.000MHZ-LY-E-T3 | MEMS OSC XO 20.0000MHZ LVCMOS | 263 More on Order |
![]() |
ASGTX-C-98.304MHZ-1-T2 | XTAL OSC VCTCXO 98.3040MHZ LVCMO | 206 More on Order |
![]() |
AX7MBF1-1216.0000C | XTAL OSC XO 1.2160GHZ CML SMD | 413 More on Order |
![]() |
AX7DBF1-1000.0000T | XTAL OSC XO 1.0000GHZ LVDS SMD | 433 More on Order |
![]() |
AX5MBF1-1800.0000C | OSC XO 1.8GHZ 2.5V CML | 383 More on Order |
![]() |
AX5MCF1-614.6400C | OSC XO 614.64MHZ 1.8V CML | 138 More on Order |
![]() |
AX7MBF1-307.6955T | XTAL OSC XO 307.6955MHZ CML SMD | 322 More on Order |
![]() |
AX5MBF1-614.6400T | OSC XO 614.64MHZ 2.5V CML | 225 More on Order |
![]() |
AX5DCF2-614.4000T | OSC XO 614.4MHZ 1.8V LVDS | 474 More on Order |
![]() |
AX5PAF2-300.0000C | OSC XO 300MHZ 3.3V LVPECL | 472 More on Order |
![]() |
AX7PAF1-74.2500C | XTAL OSC XO 74.2500MHZ LVPECL | 282 More on Order |
![]() |
ASTMHTA-12.000MHZ-AJ-E | MEMS OSC XO 12.0000MHZ LVCMOS | 363 More on Order |
![]() |
ASTMLPD-24.000MHZ-EJ-E-T | MEMS OSC XO 24.0000MHZ LVCMOS | 390 More on Order |
![]() |
ABM11W-40.0000MHZ-4-K2Z-T3 | CRYSTAL 40.0000MHZ 4PF SMD | 444 More on Order |
![]() |
ABM11W-33.0000MHZ-8-K2Z-T3 | CRYSTAL 33.0000MHZ 8PF SMD | 122 More on Order |
![]() |
ABM10W-22.1184MHZ-8-B1U-T3 | CRYSTAL 22.1184MHZ 8PF SMD | 313 More on Order |